
It is not impossible, in principle, for all 6 × 10 23 atoms in a mole of a gas to spontaneously migrate to one half of a container it is only fantastically unlikely-so unlikely that no macroscopic violation of the Second Law has ever been observed. The Second Law of Thermodynamics is statistical in nature, and therefore its reliability arises from the huge number of particles present in macroscopic systems. Thus this law is about the boundary conditions rather than the equations of motion. The existence of a thermodynamic arrow of time implies that the system is highly ordered in one time direction only, which would by definition be the "past". However, the entropy can only be a constant if the system is in the highest possible state of disorder, such as a gas that always was, and always will be, uniformly spread out in its container. If the entropy is constant in either direction of time, there would be no preferred direction. The Second Law of Thermodynamics allows for the entropy to remain the same regardless of the direction of time. 5 The arrow of time in various phenomena.2 An example of apparent irreversibility.This, however, is neither linked to the thermodynamic arrow of time, nor has anything to do with the daily experience of time irreversibility. According to the CPT theorem, this means they should also be time irreversible, and so establish an arrow of time. It would be an astronomically improbable event for all the particles to cluster together, yet the movement of any one smoke particle cannot be predicted.īy contrast, certain subatomic interactions involving the weak nuclear force violate the conservation of parity, but only very rarely. On average it would be expected that the smoke particles around a struck match would drift away from each other, diffusing throughout the available space.

It is only when the gas is studied at a macroscopic scale that the effects of entropy become noticeable. As it drifts left or right, qualitatively it looks no different. Watching a single smoke particle buffeted by air, it would not be clear if a video was playing forwards or in reverse, and, in fact, it would not be possible as the laws which apply show T-symmetry. When studying at a microscopic scale, the above judgements cannot be made. Because of the second law of thermodynamics, entropy prevents macroscopic processes showing T-symmetry. When a law of physics applies equally when time is reversed, it is said to show T-symmetry in this case, entropy is what allows one to decide if the video described above is playing forwards or in reverse as intuitively we identify that only when played forwards the entropy of the scene is increasing. Surprisingly, in either case the vast majority of the laws of physics are not broken by these processes, a notable exception being the second law of thermodynamics. A video may depict a wood fire that melts a nearby ice block, played in reverse it would show that a puddle of water turned a cloud of smoke into unburnt wood and froze itself in the process. For example, it is often very easy to tell the difference between a video being played forwards or backwards. Much like temperature, despite being an abstract concept, everyone has an intuitive sense of the effects of entropy. In thermodynamic systems that are not isolated, entropy can decrease with time, for example living systems where local entropy is reduced at the expense of an environmental increase (resulting in a net increase in entropy), the formation of typical crystals, the workings of a refrigerator and within living organisms. Thus, entropy measurement is a way of distinguishing the past from the future.
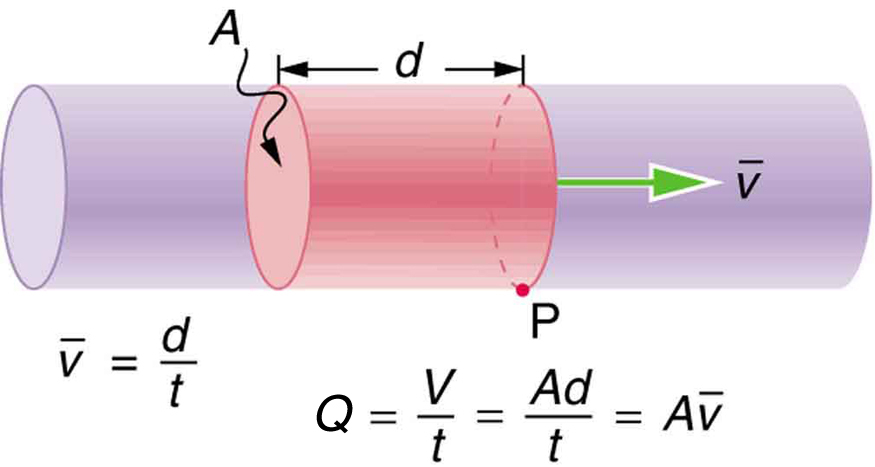
As one goes "forward" in time, the second law of thermodynamics says, the entropy of an isolated system can increase, but not decrease. Entropy is one of the few quantities in the physical sciences that require a particular direction for time, sometimes called an arrow of time.
